Translate this page into:
Three-dimensional prints from 3-dimensional cell culture aggregates of human cancer cell lines
*Corresponding author: Maddaly Ravi, Department of Human Genetics, Sri Ramachandra Institute of Higher Education and Research, Chennai, Tamil Nadu, India. mravi@sriramachandra.edu.in
-
Received: ,
Accepted: ,
How to cite this article: Ravi M, Pargaonkar A, Ramesh A, Agrawal G, Sally J, Srinivasan S, et al. Three-dimensional prints from 3-dimensional cell culture aggregates of human cancer cell lines. Sri Ramachandra J Health Sci 2021;1:10-5.
Abstract
Objectives:
Three-dimensional (3D) printing has gained significance for human health-care applications in recent years. Some of these applications include obtaining models which mimic anatomical parts. One other parallel development in the biological research area is the development of 3D cell cultures. Such cultures are now becoming the material of choice for in vitro experiments, fast replacing the traditional adherent/monolayer 2D culture approaches. We present here, a method to obtain 3D prints of 3D aggregates of three human cancer cell lines. Such 3D prints can be useful models to understand solid tumor morphologies and also as effective teaching models.
Materials and Methods:
Photomicrographs of the 3D aggregates of the human cancer cell lines SiHa, MCF-7, and A549 (human cervical cancer, breast cancer, and non-small cell lung cancer cell lines, respectively) were obtained using inverted phase contrast microscopy. Conversion of normal jpeg images into 3D files was performed using the lithophane method and CAD files obtained. The CAD files thus generated were used to print the objects using the Stratasys Polyjet J750 3D Printer.
Results:
We could obtain 3D prints of SiHa, MCF-7, and A549 (human cervical cancer, breast cancer, and non-small cell lung cancer cell lines, respectively) 3D aggregates/spheroids.
Conclusion:
It is hoped that this approach will be useful for studying solid tumor morphologies in finer details. Furthermore, other benefits of such 3D prints would be in them being excellent models for teaching purposes.
Keywords
Cancer cell lines
3-dimensional cultures
3-dimensional printing
Solid tumor models
Cancer research
INTRODUCTION
There has been an increasing trend in the recent years to develop advanced in vitro systems that mimic in vivo conditions as can be used for translational research. Furthermore, there is an increasing trend to utilize human cells in vitro (both established cell lines and primary cultures) in dynamic conditions which closely resemble in vivo physiology. This is more due to the concerns regarding the animal usage for research and also the ways in which animal models differ from human physiology. One major milestone in this regard is the introduction of 3-dimensional (3D) cultures where cells no longer are grown as attached or adherent cells. This proved important for better understanding cellular physiology and also for translating basic research to long-term clinical applications.[1]
The collaboration among various technologies has enabled generating newer tools for basic and translational research. An example of a result of such collaboration is the advent of 3D printing technologies for medical and biomedical application. Once largely restricted to the realm of engineering sciences such as the automobile industry, additive printing has fast gained momentum for human health-care applications. These applications include for both diagnostic purposes and for therapeutic interventions and appropriately referred to as 3D bioprinting.
3D bioprinting is a biofabrication approach that utilizes conventional 3D printing techniques to create artificial tissues or organs by printing and arranging biomaterials in a layer-by-layer configuration.[2,3] Bioinks have the preferred physical and chemical properties that help in the development of biological constructs, which are functionally relevant, biocompatible, biodegradable, and are able to appropriately mimic biological tissues. [3,4] The most common types of bioinks used as scaffolds are hydrogel based and consist of those that are proteins (collagen and gelatin), polysaccharides (alginate and agarose), decellularized extracellular membranes (Matrigel), and synthetic polymer-based (PEG) bioinks. These bioinks can also be mixed with certain biomolecules, such as growth factors that encourage cell differentiation and proliferation, and blood plasma, which is an amalgam of essential minerals and proteins. 3D bioprinting offers enormous versatility and can be applied to microfluidics, cancer research (cancer initiation and progression), drug testing, examining tissue pathology pertaining to various diseases, and high-throughput assays.[2,4,5]
It has been well documented in the literature that 3D aggregates of cancer cell lines show fundamental differences in a variety of aspects compared to their 2D culture counterparts. One striking feature of the cell line grown in 3D condition is in them spontaneously acquiring distinct morphological shapes comprising of cell aggregates. Furthermore, it has been observed/ demonstrated that different human cancer cell lines from 3D aggregate with different morphological characteristics. Fundamental genetic aspects of the cancer cell lines and other physiological mechanisms have been identified as contributing factors to such morphological differences of the 3D aggregates of various cell lines representing different cancer types. [6] Studying the gross morphological aspects of 3D aggregates such as the mass, size, and volume has become a new study endpoint for in vitro experiments in the recent years.[7]
We have developed 3D prints of spheroids and aggregates obtained from three different cell lines which were grown in 3D conditions. We believe such 3D prints will be invaluable for studying solid tumor morphologies apart from being excellent models for educational purposes. To the best of our knowledge, we have not come across any other study which has reported developing 3D prints of human cancer cell line 3D aggregates, although several studies have emphasized on the importance of the morphological aspects of such 3D aggregates.
MATERIALS AND METHODS
Establishment of 3D cultures
Adherent monolayers of MCF-7, A549, and SiHa cell lines were maintained in Dulbecco’s Modified Eagle Medium (DMEM) supplemented with 10% fetal bovine serum according to standard protocols. The 3D spheroids and aggregates of these cell lines were obtained by culturing them on 1% agarose hydrogels. About 1% agarose gel was prepared by melting the required amounts of powdered agarose (80oC) in plain DMEM. Precautions were taken to not boil the mixture and 1 mL of molten agarose was added in the wells of a 12-well plate. The plates were exposed to UV light in a laminar air flow for 60 min which for rendering the gel free of contamination and also for efficient polymerization of the gel.
The monolayers of MCF-7, A549, and SiHa were harvested by typsinization and checked for viability before seeding them into the wells containing the agarose hydrogels. The seeding densities used for MCF-7, A549, and SiHa were 0.15 × 106 cells/ml, 0.169 × 106 cells/ml, and 0.96 × 106 cells/ ml, respectively, for the 12-well plates. The developing 3D spheroids and aggregates were observed at 24 h intervals and photomicrographs were obtained at the exponential cell culture phase stage.
Conversion of images to 3D printable format
Conversion of normal jpeg images into 3D files was performed using the lithophane method. The photomicrographs (jpeg images) of the 3D aggregates were used to create.stl files which were converted as.vrml file using the Ultimaker CURA software version 4.4.1. Colors were incorporated into the.vrml files using the software Magics with which we also recreated the texture as were originally found on the 3D images. These files were used to obtain to color data to get. vrml or.obj output which are the color printing formats. The entire file conversion process is presented in Figure 1. The net image outputs after the conversion of the jpeg images of the spheroids of the three cell lines into printable format are presented in Figures 2-4.
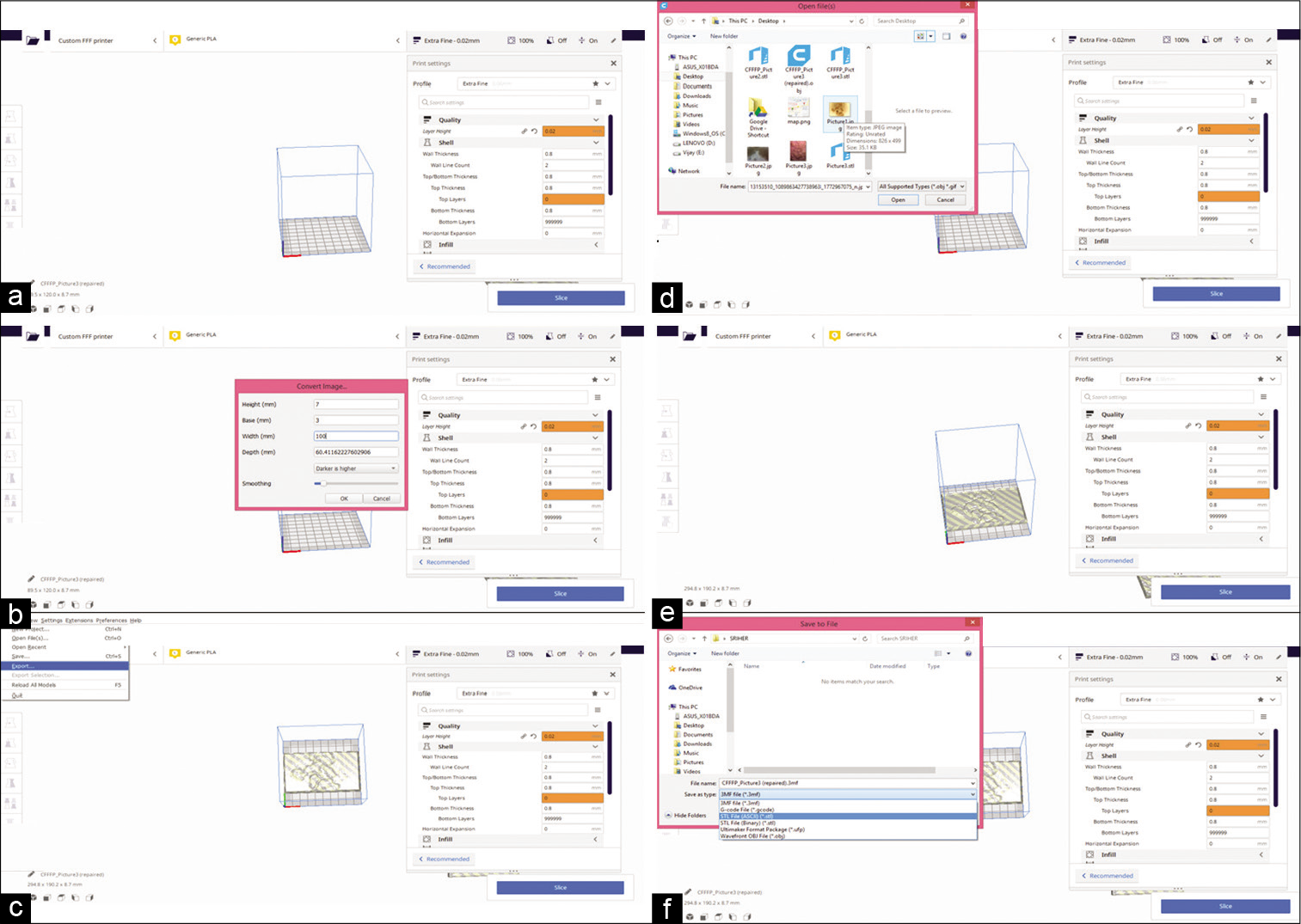
- The conversion process of the jpeg images from the photomicrographs was converted into.stl format by the series of steps as presented in the panels (a-f). The Ultimaker CURA software version 4.4.1 was used for the conversion.
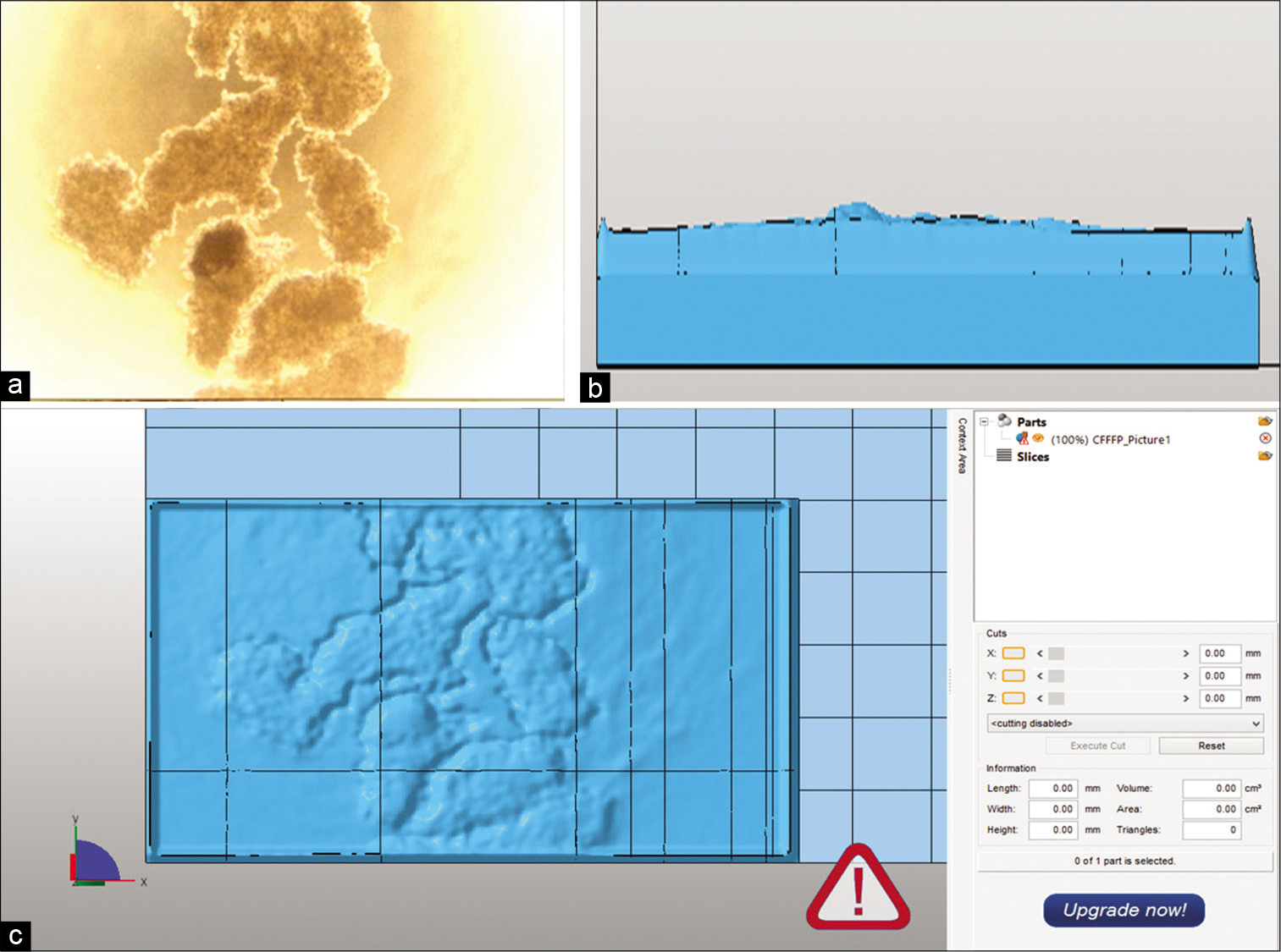
- The photomicrograph and the vrml file obtained from the photomicrograph as the final image to be 3D printed for the SiHa cell line. The panels (a-c) show the 3D aggregates, the side view of the image created and the surface view of the created image, respectively
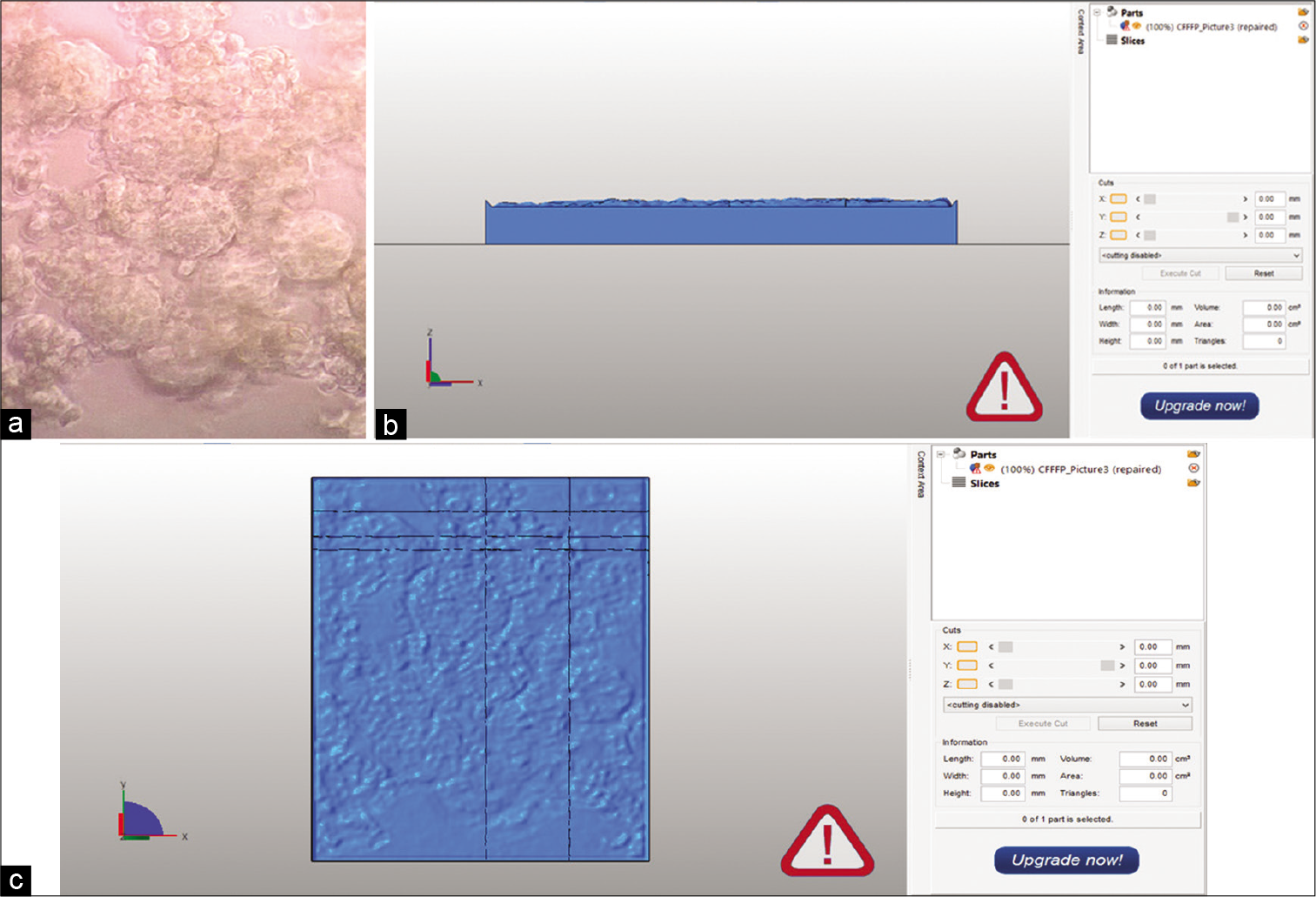
- The photomicrograph and the vrml file obtained from the photomicrograph as the final image to be 3D printed for the MCF-7 cell line. The panels (a-c) show the 3D aggregates, the side view of the image created, and the surface view of the created image, respectively
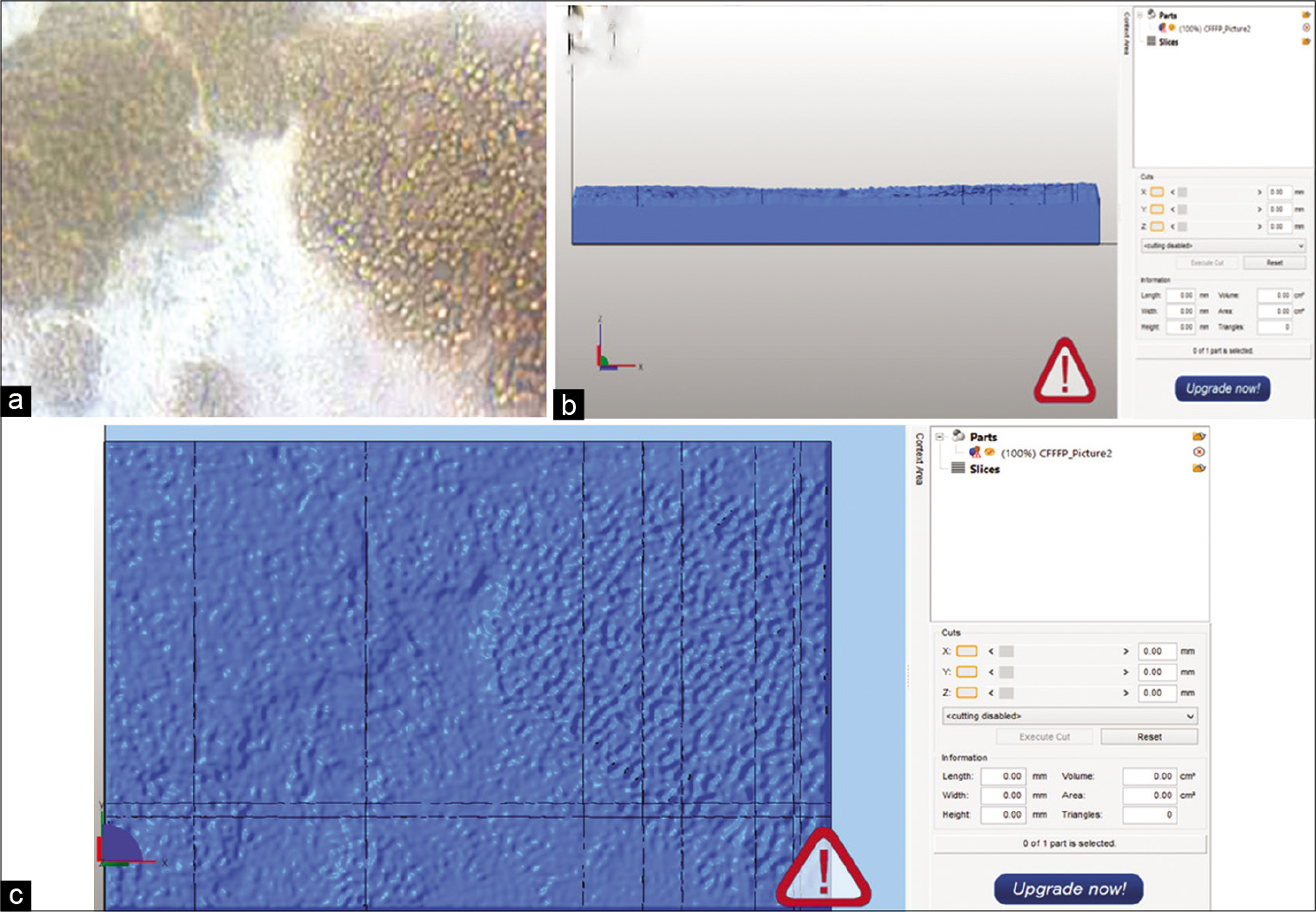
- The photomicrograph and the vrml file obtained from the photomicrograph as the final image to be 3D printed for the A549 cell line. The panels (a-c) show the 3D aggregates, the side view of the image created, and the surface view of the created image, respectively.
Obtaining 3D prints
The CAD files thus generated were used to print the objects using the Stratasys Polyjet J750 3D Printer. We printed multiple parts in single build to show different color capability in one go. The information regarding printing steps are presented in Table 1.
Tray estimations | ||
---|---|---|
1 | High quality | High mix |
Print time | 3 h 6 min | 1 h 37 min |
Total materials (g) | 227 | 215 |
Total support (g) | 66 | 32 |
VeroBlackPlus | 6 | 3 |
VeroClear | 6 | 3 |
VeroCyan | 9 | 6 |
VeroMagenta | 14 | 11 |
VeroPureWhite | 177 | 180 |
VeroYellow | 15 | 12 |
FullCure705 | 66 | 32 |
RESULTS
3D prints of the three cell lines aggregates or spheroids were obtained through a series of steps. The final 3D prints of the three types of spheroids are presented in Figure 5. The overall scheme of arriving at the 3D prints from the photomicrographs is illustrated in Figure 6.
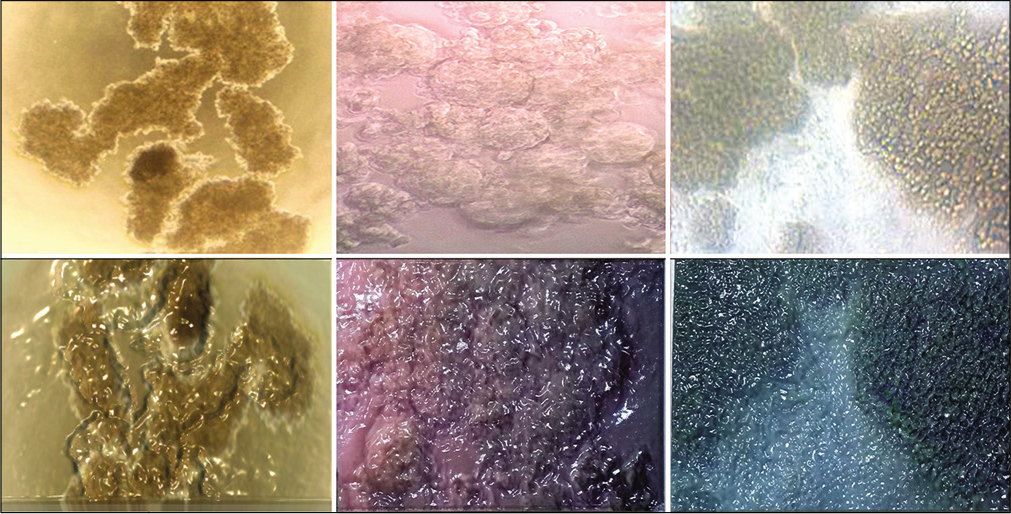
- The 3D aggregates and the 3D prints are presented for the three cell lines, the SiHa, the MCF-7, and the A-549. The morphological features were quite replicated in the 3D prints making them a permanent record of the spheroids/aggregates at a much larger scale (size).
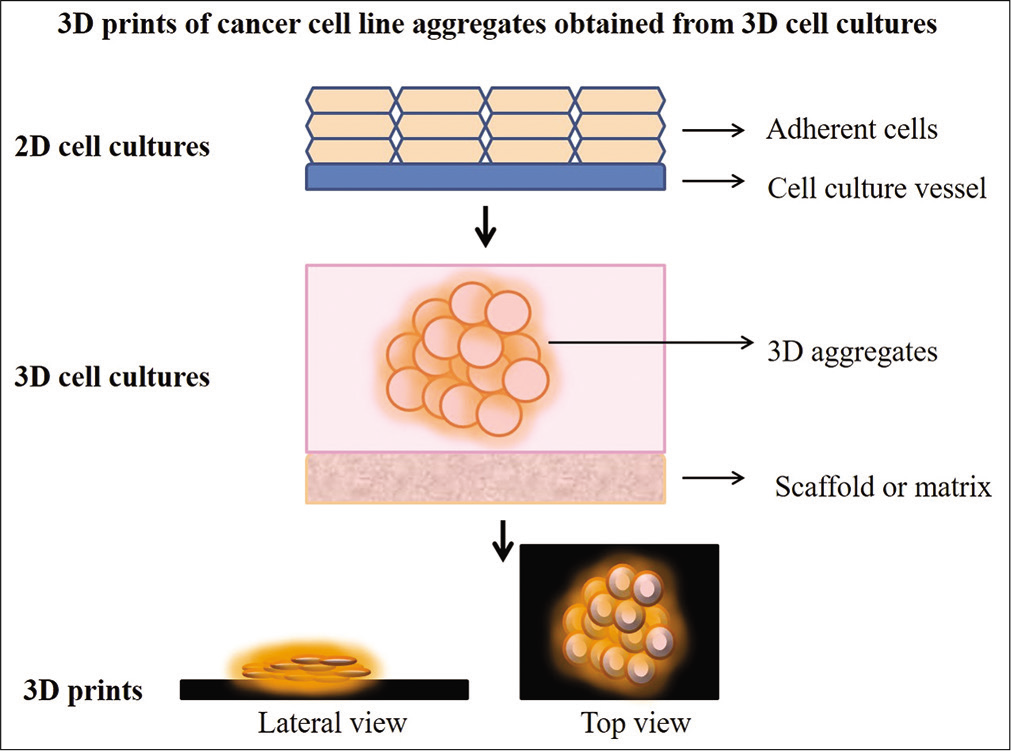
- The overall scheme of study and the steps involved are illustrated. The steps begin with obtaining CAD files from the photomicrographs and the final result is obtaining the 3D prints.
DISCUSSION
3D cell cultures have gained significant importance as useful research material, especially for cancer research and drug discovery applications. 3D cultures of cancer cell lines differ in several ways compared to their 2D counterparts. One immediate and remarkable difference as can be observed in such 3D cultures is the spontaneous morphologies that these cultures exhibit. It is interesting to note that different cell lines representing various human cancer types do not form 3D aggregates with similar morphologies. Rather, various cancer cell lines exhibit different 3D aggregate morphologies such as the stellate, grape-like, solid mass, diffused, and ascinars. The formation of such aggregates spontaneously is known to have a definite physiological (genetic) basis as specific for the different cancer cell types. Thus, studying the gross morphological aspects of 3D aggregates such as the mass, size, and volume has become a new study endpoint for in vitro experiments in the recent years. Furthermore, it is for the same reasons as mentioned that the 3D aggregates obtained from single cell lines are useful in vitro models for a variety of endpoints including understanding basic cancer cell physiology and for drug discovery.
Homogenous cultures of single-cell type have been used widely for research and probably the most versatile of the in vitro experimental material available. This is augmented by the flexibility such cultures offer for including a number of experimental variables. Thus, 3D aggregates of a single cell type have their own niche in vitro experiments. 3D printing of such cultures has potentials to throw more light of the morphological aspects of solid tumors. The methodology described here is straight forward and is a model one which can be used or modified for finer applications. For example, instead of using the phase-contrast microscope images as the starting point, confocal images with differentially stained cellular components can be used as the initial material from which the 3D prints can be obtained. Similarly, the method described can be used to obtain 3D printed models of cocultures aggregates or organotypic cultures using suitable inks or dyes. The extent to which and the ways in which the 3D prints mimic natural solid tumors has not been attempted in this study and will be a useful approach for further exploration. The applications of the approach described include one more dimension to better understanding solid tumor morphology and for obtaining efficient teaching models.
CONCLUSION
A striking feature of cancer cell lines grown as 3D culture is their spontaneous acquisition of distinct morphological structures. These structures which are referred to as the aggregates or spheroids are known to be of various types and also cell-line specific. Hence, studying the morphologies of 3D cultures of human cancer cell lines will enable one to decipher inherent physiological aspects of solid tumors. Here we present a straight-forward method to obtain 3D prints of a few human cancer cell line 3D aggregates. We hope that this approach will be useful for studying solid tumor morphologies in finer details. Also, such 3D prints will provide us with excellent teaching models similar to the currently available anatomical models that are used for academic purposes.
Acknowledgments
The work described in this manuscript is not a part of any funded project and did not receive any funding.
Declaration of patient consent
Patient’s consent not required as there are no patients in this study.
Financial support and sponsorship
Nil.
Conflicts of interest
There are no conflicts of interest.
References
- 3D models in the new era of immune oncology: Focus on T cells, CAF and ECM. J Exp Clin Cancer Res. 2019;38:117.
- [CrossRef] [PubMed] [Google Scholar]
- Progress in 3D bioprinting technology for tissue/organ regenerative engineering. Biomaterials. 2020;226:119536.
- [CrossRef] [PubMed] [Google Scholar]
- Three-dimensional printing of biological matters. J Sci Adv Mater Device. 2016;1:1-17.
- [CrossRef] [Google Scholar]
- Bioinks for 3D bioprinting: An overview. Biomater Sci. 2018;6:915-46.
- [CrossRef] [PubMed] [Google Scholar]
- Recent advances on utilization of bioprinting for tumor modeling. Bioprinting. 2020;18:10.
- [CrossRef] [PubMed] [Google Scholar]
- The morphologies of breast cancer cell lines in three-dimensional assays correlate with their profiles of gene expression. Mol Oncol. 2007;1:84-96.
- [CrossRef] [PubMed] [Google Scholar]
- The volume of three-dimensional cultures of cancer cells in vitro influences transcriptional profile differences and similarities with monolayer cultures and xenografted tumors. Neoplasia. 2017;19:695-706.
- [CrossRef] [PubMed] [Google Scholar]